Introduction
POS is a rare clinical entity characterized by positional dyspnea (platypnea) and measurable drop in arterial desaturation (orthodeoxia) of >5% or a PaO2 >4 mmHg, from supine to an upright position.1 It was first described in 1949 by Burchell et al, but not only until 1969 and 1978, Altman et al and then Robin et al, coined the terms “platypnea” and “orthodeoxia” respectively.2–4
The mechanisms underlying this clinical syndrome remain unclear after more than 70 years, and now, with new cases being reported in the context of severe SARS-CoV2 illness, clinicians are formulating new hypotheses to explain this enigmatic condition.
The hypoxia and arterial desaturation in this syndrome are explained by the mixing of deoxygenated venous blood with oxygenated arterial blood via shunt. According to a review of literature conducted by Agrawal et al from January 1949 up to November 2016, depending on the site of the shunt, POS can be classified as intracardiac, extracardiac, or due to miscellaneous etiologies.1
The intracardiac shunt is the predominant etiology, with right-to-left shunting through a Patent Foramen Ovale (PFO) being the most prevalent cause of POS (followed by atrial septal defects and atrial septal aneurysms). However, these intracardiac abnormalities are congenital defects, so there must be secondary physiological and/or anatomical changes that cause POS later in life.5
Extracardiac shunts are less frequent, with the lung being the most commonly affected site. In these cases, POS is assumed to be caused by ventilation/perfusion mismatches in the context of pulmonary arteriovenous malformations, COPD, hepatopulmonary syndrome and interstitial lung disease, among others.1,6
The last group includes several etiologies which lead to POS through unexplained mechanisms, from autonomic neuropathy to lung toxicity caused by radiation, amiodarone, or organophosphorus poisoning.
We present a recent case of POS that occurred in our institution, aiming to describe the clinical characteristics and outcome, as well as to discuss the pathophysiologic mechanism of intracardiac POS in patients with severe SARS-CoV-2 interstitial pneumonia.
Case Presentation
An 80-year-old male was transferred to our hospital on 12/01/2021 with fever, shortness of breath, confusion, diarrhea and vomiting with uncertain onset, who had tested positive for SARS-CoV2 on 05/01/2021. The patient had a history of ischemic heart disease with preserved ejection fraction and controlled hypertension; was a non-smoker, and had no previous history of pulmonary or congenital heart disease.
On admission to our ward, the patient was apyretic and disoriented. His blood pressure was 136/64 mmHg and his pulse rate was 82 bpm. His oxygen saturation was 96% with supplemental oxygen (2 L/min through nasal cannula). Respiratory examination showed tachypnea, but with no use of accessory muscles of respiration, chest expansion was symmetrical. There were wheezes in the medium third of the right hemithorax and crackles in the right pulmonary base. The remaining systemic examination was within normal limits, including the cardiovascular system. Arterial blood gas analysis on room air confirmed type 1 respiratory failure (pH 7.51; PaO2 60 mmHg; paCO2 26 mmHg, R P/F 288). Complete blood count showed leukocytosis (11200/ul) and mild lymphopenia (750/ul), with C-reactive protein elevation (62 mg/dL). Serum creatinine was within normal limits. The liver panel showed slight cytolysis (AST 95 U/L and ALT 95 U/L). There was severe hyponatremia (serum Na+ 102mmol/L), due to thiazide use (usual medication of the patient) in association with the gastrointestinal symptoms (vomiting and diarrhea). His Pro-BNP levels were 480 pg/mL.
The patient was managed as a case of severe COVID-19 disease, as per protocol (supplemental oxygen therapy, steroids, anticoagulation, self-prone and early kinesiotherapy). Adequate correction of hyponatremia was achieved through fluid therapy and withdrawal of causative drugs.
On the 12th day of illness, there was a deterioration of respiratory failure, with the need to escalate oxygen therapy to high flow nasal cannula (HFNC) and non-invasive ventilation. A chest CT angiogram was negative for pulmonary embolism, but showed bilateral diffuse peripheral ground-glass opacity, coexisting with interstitial consolidation in lower zones. (Fig. 1). There were no signs of fluid overload or bacterial infection. Because of the persistence of respiratory failure, the chest CT angiogram was repeated on the 21st day of illness, showing fibrotic evolution and organizing appearance, suggesting complication with organizing pneumonia (Fig. 2). On the 30th day of illness, and the 23rd day of high-dose steroid therapy (1mg/kg of prednisolone), there was only a slight clinical improvement; it was then, with further patient mobilization that it was noticed that his hypoxemia was more profound while he was standing or sitting (with the need to escalate from nasal cannula (estimated FiO2 31%) up to high flow oxygen mask (estimated FiO2 80%)), and improved when he laid down. His saturation decreased from 96% in the recumbent position to 88% in orthostasis.
Since cardiac-related POS is the most reported etiology, a transthoracic echocardiogram (TTE) with bubble contrast with intravenous agitated saline was performed. The study showed normal-sized chambers, no significant valvular heart disease, inferior vena cava caliber in the upper normal limit (21mm). Pulmonary arterial flux suggested pulmonary hypertension (it was not possible to estimate pulmonary systolic arterial pressure) and a significant right-to-left shunt through a presumed PFO was found - the appearance of bubbles in the left atrium within 3 cardiac cycles suggesting an intracardiac shunt. (Fig. 3)7,8
Therefore, the diagnosis of POS with intracardiac shunt was made. It was assumed extensive post-COVID-19 organizing pneumonia, precipitated pulmonary hypertension and reversion of shunt from left-to-right to right-to-left (elevated right atrial pressure).
The definitive treatment of POS due to intracardiac shunt usually requires repair of the cardiac anomaly. However, the functional defects must also be approached by correcting the underlying etiology. After discussion with Cardiology, it was decided that the timing was not ideal to correct the primary cardiac abnormality, and a watchful waiting approach was instituted: waiting for the resolution of interstitial damage and consequently, pulmonary hypertension. Under corticosteroids and physiotherapy, platypnea-orthodeoxia improved by day 45 of illness. On discharge, the patient denied dyspnea and his PaO2 was 69.6mmHg on room air and orthostatic position. The patient was discharged on steroid weaning and with indication to maintain physical rehabilitation.
Discussion
Although there isn´t a definitive explanatory mechanism for POS, it is commonly accepted that pre-existent interatrial communication requires an additional anatomical or functional abnormality to produce shunting.1,5,8 Although the estimated prevalence of PFO is 25 to 30% in the general population,9 most people with this septal defect never develop POS. In fact, the majority of individuals with an isolated PFO do not have right-to-left shunt because the left atrial pressure is 5-8 mmHg higher than the right atrial pressure, allowing a functional closure.
It is not only until the right atrial pressure exceeds the left atrial pressure, by any insult that increases right atrial pressure – pulmonary embolism, right myocardial infarction, hydrothorax, pneumothorax, acute pericardial effusion, or pulmonary hypertension, among others - that the pressure gradient reverses, leading to right-to-left shunting.10 So, what caused gradient reversal in our patient and why did this congenital defect only manifest itself at an elderly age?
SARS-CoV-2 pneumonia has been characterized for leading to bilateral ground-glass opacities in a peripheral distribution, predominantly involving the lower lobes.11,12 The positional change from recumbent to upright leads to gravitational redistribution of blood flow. On one hand, this leads to an increased unmatched basal pulmonary blood flow: the blood is redirected to poorly ventilated parts with severe derangements due to interstitial pneumonia´s fibrotic evolution. On the other hand, orthostasis reduces the right ventricular preload decreasing the output to pulmonary arteries, leading to alveolar pressure exceeding pulmonary arterial and venous pressures at the apexes, and thus increasing the alveolar hypoventilation on the non-dependent zone blood flow (known as zone 1 phenomenon). Zone 1 phenomenon is aggravated due to COVID-19 related procoagulant state12,13 vasoplegia and microthrombi.14–16 Consequently, reduced lung compliance due to pulmonary fibrosis enhanced by this procoagulant state culminates in pulmonary hypertension causing transient elevation of right atrial pressure, reversing the shunt and leading to the mix of deoxygenated venous blood with oxygenated arterial blood in the left atrium -causing both orthodeoxia and platypnea (Fig. 4).
Although the underlying mechanisms of POS in the context of severe SARS-CoV-2 pneumonia have rarely been documented, according to the most recent reported cases,5,7,12,14 these are the proposed pathophysiological mechanisms for POS in severe COVID-19 illness (even in the absence of an intracardiac shunt), with basal parenchymal disease leading to intra-pulmonary anatomical or physiological shunt.14
It remains unclear why these perfusion/ventilation mismatches induced by postural exchange do not occur in other interstitial lung diseases.
Hence, POS is an under-recognized clinical feature in severe SARS-CoV-2 pneumonia, and in general, because supine and orthostatic O2 saturations are not routinely collected in the acute phase. There are little data about the outcomes of patients with inter-atrial defects who develop COVID-19 3 which suggests that these congenital malformations are being underdiagnosed. This represents a hindrance since, according to literature, critically ill patients with inter-atrial shunts have worse outcomes, they respond poorly to positive expiratory pressure, are ventilated longer, and have longer admissions.17,18
Conclusion
POS should be considered in COVID-19 pneumonia patients after ruling out other causes of respiratory distress, such as secondary respiratory infection, pulmonary embolism, or heart failure. It is paramount to determine the etiology, since management should be guided by the pathophysiology of the shunt, allowing targeted treatment and symptom alleviation, and enabling the rehabilitation process. Further investigation should be led, to enlighten the still unknown mechanisms of POS, and also to raise awareness about this entity in severe COVID-19 survivors, improving the outcomes and reducing morbidity.
Figura I
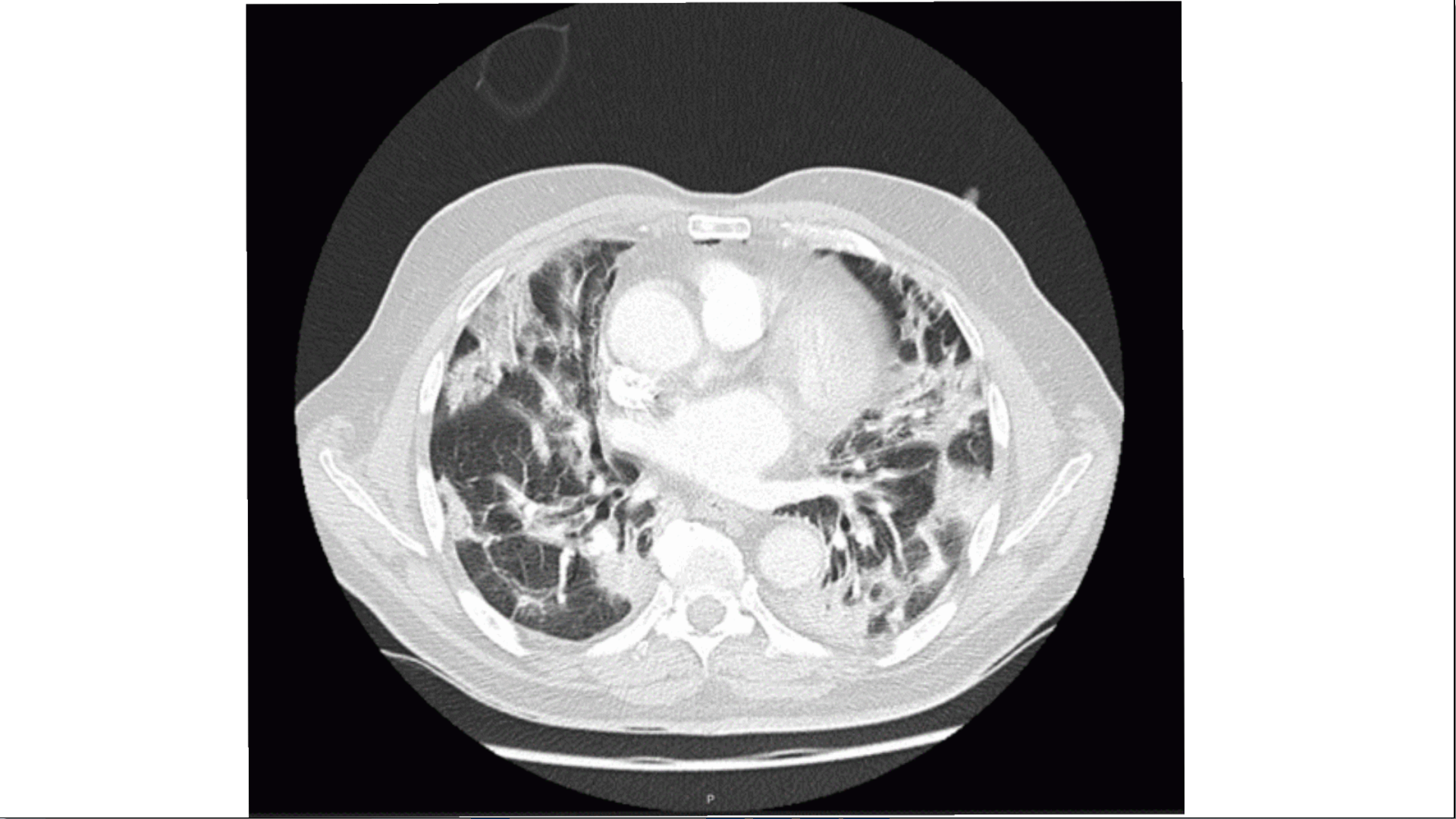
Bilateral ground-glass opacities with patchy distribution, coexisting with multifocal consolidations predominantly in posterior subpleural topography of the lower lobes (CT on 17th January 2021)
Figura II
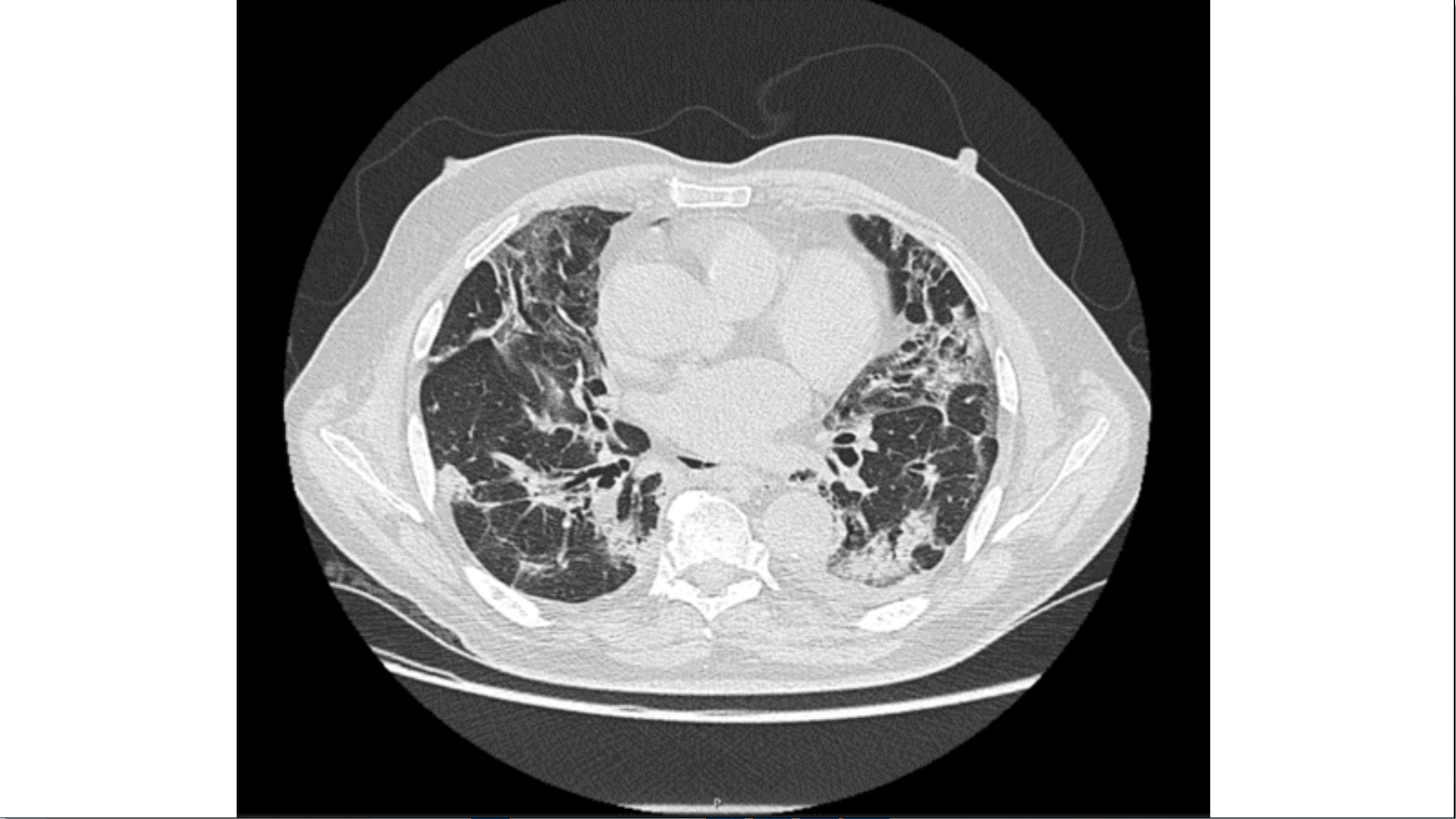
Fibrotic evolution of interstitial pneumonia. Decreasing ground-glass opacities with peribronchial consolidating areas of organized appearance with traction bronchiectasis. (CT on 26th January 2021)
Figura III
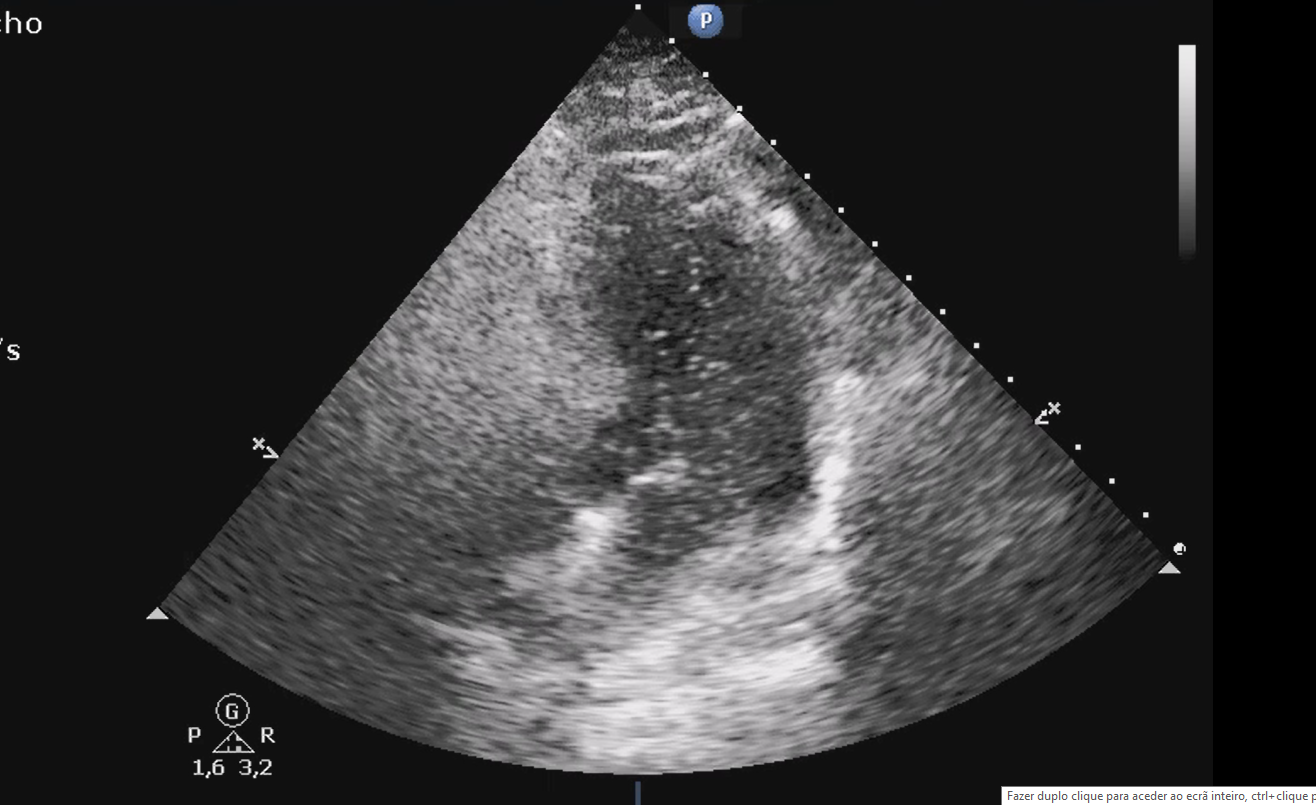
TTE view of a positive bubble study with right-to-left bubble shunting through PFO. Right chambers (RV, RA) full of agitated saline contrast and bubbles seen in the left chambers (LA, LV)
Figura IV
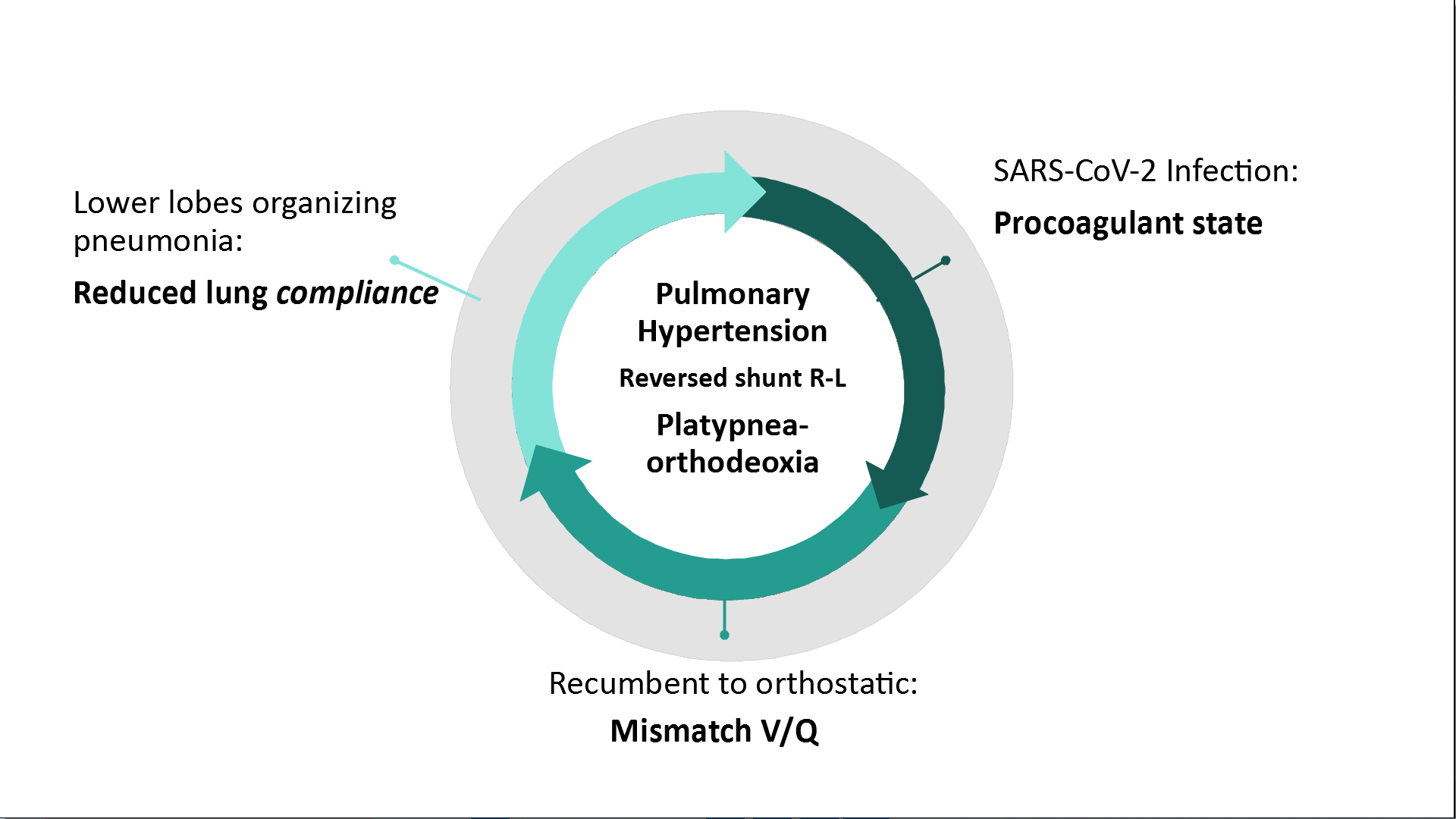
Fisiopathology of POS in SARS-CoV-2 Pneumonia
BIBLIOGRAFIA
1. Agrawal A, Palkar A, Talwar A. The multiple dimensions of Platypnea-Orthodeoxia syndrome : A review. Respir Med [Internet]. 2017;129:31–8. Available from: http://dx.doi.org/10.1016/j.rmed.2017.05.016
2. Burchell, H.B.H.H.J.; Wood EH. Reflex orthostatic dyspnea associated with pulmonary hypotension. Am J Physiol. 1949;159(Reflex orthostatic dyspnea associated with pulmonary hypotension):563–4.
3. Altman M, Robin ED. Platypnea (Diffuse Zone I Phenomenon?). N Engl J Med. 1969;281(24):1347–8.
4. Robin ED, Laman D, Horn BR, Theodore J. Platypnea Related to Orthodeoxia Caused by True Vascular Lung Shunts. N Engl J Med. 1976 Apr 22;294(17):941–3.
5. Yepes I, Ji S, Wu F, Tijmes S, Roberts J. Platypnea-Orthodeoxia Syndrome: Rare or Under-Diagnosed Syndrome? 3 Case Reports and a Literature Review. Cardiovasc Revascularization Med. 2021 Jan 1;22:115–9.
6. Gattinoni L, Coppola S, Cressoni M, Busana M, Rossi S, Chiumello D. COVID-19 does not lead to a “typical” acute respiratory distress syndrome [Internet]. Vol. 201, American Journal of Respiratory and Critical Care Medicine. American Thoracic Society; 2020 [cited 2021 Mar 7]. p. 1299–300. Available from: /pmc/articles/PMC7233352/
7. Singh K, Kadnur H, Ray A, Khanna P, Singh A, Wig N, et al. Platypnea-orthodeoxia in a patient with severe COVID-19 pneumonia. Monaldi Arch Chest Dis [Internet]. 2020 Dec 23 [cited 2021 Mar 7];90(4):718–20. Available from: https://pubmed.ncbi.nlm.nih.gov/33372742/
8. Cheng TO. Mechanisms of platypnea-orthodeoxia: what causes water to flow uphill? Vol. 105, Circulation. 2002.
9. Homma S, Messé SR, Rundek T, Sun YP, Franke J, Davidson K, et al. Patent foramen ovale. Nat Rev Dis Prim [Internet]. 2016 Jan 21 [cited 2021 Mar 7];2(1):1–15. Available from: https://www.nature.com/articles/nrdp201586
10. Rajendram R, Kharal GA, Mahmood N, Puri R, Kharal M. Rethinking the respiratory paradigm of COVID-19: a ‘hole’ in the argument [Internet]. Vol. 46, Intensive Care Medicine. Springer; 2020 [cited 2021 Mar 7]. p. 1496–7. Available from: https://pubmed.ncbi.nlm.nih.gov/32488341/
11. Wang Y, Dong C, Hu Y, Li C, Ren Q, Zhang X, et al. Temporal Changes of CT Findings in 90 Patients with COVID-19 Pneumonia: A Longitudinal Study. Radiology [Internet]. 2020 Aug 1 [cited 2021 Mar 7];296(2):E55–64. Available from: /pmc/articles/PMC7233482/
12. Longo C, Ruffini L, Zanoni N, Longo F, Accogli R, Graziani T, et al. Platypnea-orthodeoxia after fibrotic evolution of SARS-CoV-2 interstitial pneumonia. A case report. Acta Biomed [Internet]. 2020 Sep 11 [cited 2021 Mar 7];91(3):1–7. Available from: https://pubmed.ncbi.nlm.nih.gov/32921749/
13. Fox SE, Akmatbekov A, Harbert JL, Li G, Quincy Brown J, Vander Heide RS. Pulmonary and cardiac pathology in African American patients with COVID-19: an autopsy series from New Orleans. Lancet Respir Med [Internet]. 2020 Jul 1 [cited 2021 Mar 7];8(7):681–6. Available from: /pmc/articles/PMC7255143/
14. Tan GP, Ho S, Fan BE, Chotirmall SH, Tan CH, Lew SJW, et al. Reversible platypnea-orthodeoxia in COVID-19 acute respiratory distress syndrome survivors. Respir Physiol Neurobiol. 2020 Nov 1;282:103515.
15. Hert R, Albert RK. Sequelae of the adult respiratory distress syndrome [Internet]. Vol. 49, Thorax. BMJ Publishing Group; 1994 [cited 2021 Mar 7]. p. 8–13. Available from: https://pubmed.ncbi.nlm.nih.gov/8153946/
16. Radermacher P, Maggiore SM, Mercat A. Gas exchange in acute respiratory distress syndrome [Internet]. Vol. 196, American Journal of Respiratory and Critical Care Medicine. American Thoracic Society; 2017 [cited 2021 Mar 7]. p. 964–84. Available from: https://pubmed.ncbi.nlm.nih.gov/28406724/
17. Mekontso Dessap A, Boissier F, Leon R, Carreira S, Roche Campo F, Lemaire F, et al. Prevalence and prognosis of shunting across patent foramen ovale during acute respiratory distress syndrome*. Crit Care Med [Internet]. 2010 Sep [cited 2021 Mar 7];38(9):1786–92. Available from: http://journals.lww.com/00003246-201009000-00003
18. Legras A, Caille A, Begot E, Lhéritier G, Lherm T, Mathonnet A, et al. Acute respiratory distress syndrome (ARDS)-associated acute cor pulmonale and patent foramen ovale: a multicenter noninvasive hemodynamic study Philippe Vignon 4,5,12 and on behalf of the ARCO and CRICS network. 2015;